Environmental Modules
Greening Across the Chemistry Curriculum English | Versión en Español
| Versão em Português (Brasil) 
A Green Chemistry Module
Suggested Use: An environmental chemistry course during a discussion of pesticides and/or water pollution.
ANTIFOULANTS (Marine Pesticides)
Michael C. Cann, Chemistry Department, University of Scranton
Pesticides
Unwanted organisms are killed or otherwise controlled by pesticides. Chemical pesticides usually act by interfering with a bodily function in the target organism(s). Pesticides are used to control a wide range of organisms with some of the most common being insects (insecticides), plants (herbicides) and microorganisms (disinfectants). Other pesticides are used to control algae (algicides), rodents (rodenticides), fish (pisicides), fungi (fungicides), snails and slugs (molluscicides) and birds (avicides). Some of the most well known chemical pesticides include the organochlorine insecticides (e.g. DDT, mirex, dieldrin and aldrin), the organophosphate insecticides (e.g. malathion, parathion, chlorpyrifos) and the chlorophenol derived herbicides (e.g. 2,4-D and 2,4,5-T).
The Growth of Soft (algae and seaweed) and Hard (barnacles) Foulants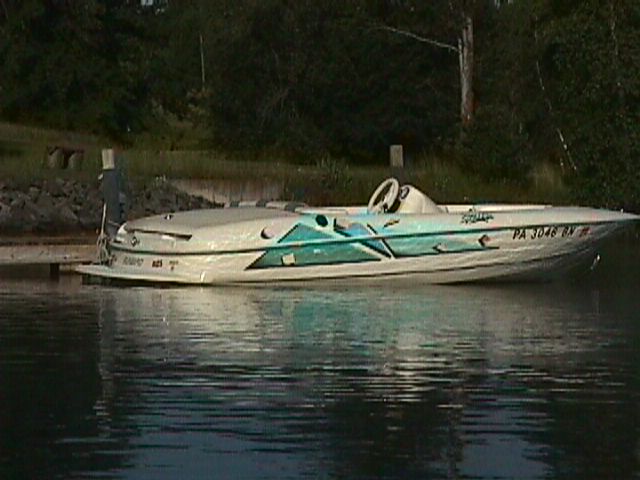
I am fortunate to live on a little lake in northeastern Pennsylvania and with the passage of winter and the arrival of warm weather in the spring, the annual launching of our small boat takes place. The first spin around the lake is always the fastest and as the summer fades into fall the top speed of our boat declines by 2-3 miles/hour. As we try to stretch the boating season we at last succumb to the chill of late fall and pull the boat out for the winter. Before storing the boat we always clean it, inside and out. The amount of slime that builds up on the hull that is beneath the waterline never ceases to amaze me and requires significant elbow grease and cleaner to restore the hull to its original brilliance. This buildup of slime leads to increased drag on the boat and in part the decrease in the top speed. In fact a layer of slime only 1 mm thick on a boat's hull can increase the drag 80% and a loss in speed of 15%.1
Anyone who has ever been to the ocean has seen rocks or dock pilings covered with algae and seaweed (soft foulants) and encrusted with barnacles and diatoms (hard foulants). Of course the hull of a ship is constantly immersed in water for several years and the buildup of these same marine organisms can lead to a significant hydrodynamic drag on the ship. This drag on a ship impedes its passage through the water resulting in increased fuel usage, causing higher operational and environmental costs. It has been estimated that it would cost the shipping industry $3 billion in added fuel costs if the hulls of ships were left untreated.2 Additional costs occur when the ship is placed in dry dock. These include the cost to clean the hull and the down time, which are estimated at $2.7 billion annually. In addition, environmental costs not only result from increased consumption of fossil fuels (a nonrenewable resource) but also increased amounts of carbon dioxide (a greenhouse gas) and other atmospheric pollutants (nitrogen oxides, sulfur oxides, unburned hydrocarbons, ozone etc.).
The Use of Antifoulants (Pesticides) to Control the Growth of Marine Organisms
Organotin Antifoulants
In order to inhibit the attachment and growth of marine organisms, the hulls of ships are usually treated with chemical compounds. These compounds, known as antifoulants are generally dispersed in the paint as it is applied to the hull. Organotin compounds particularly tributyltin oxide (TBTO), are very effective as antifoulants and have been the antifoulant of choice for many years (organotins are also widely used in agricultural, wood and plastics industries). The effectiveness of TBTO is a result of the fact that it gradually leaches from the hull killing the fouling organisms in the surrounding area.
Although organotin compounds are effective antifoulants and have saved the shipping industry billions of dollars by reducing fuel consumption and reducing dry dock time, in the 1980’s it was recognized that TBTO and other organotin antifoulants have relatively long half-lives in the environment (half-life of TBTO in seawater is > 6 months).1 Thus significant concentrations of these compounds can be found in ocean sediments as well as the water, and they tend to bioconcentrate in marine organisms. As a result of bioconcentration it is not uncommon for the concentration of TBTO in marine organisms to be 104 times greater than in the surrounding water.
Not only are oganotins persistent pollutants, but they are also chronically toxic to marine life at the ppt level and may enter the food chain. It has been shown in particular that TBTO causes deformations in the thickness of oyster shells, sex changes in whelks and imposex in snails. In addition the immune systems in dolphins, fish and other marine organisms may be compromised as a result of the bioconcentration of oranonotin compounds. Because of these environmental problems and concerns, organotin antifoulants are being phased out globally,3 with the IMO(International Maritime Organization) in the process of banning the application of organotins as antifouling agents as of January 1, 2003. Japan has already banned the use of organotin antifoulants and in the United States the use of tributyltin antifoulants has been severely restricted by the Organotin Antifouling Paint Control Act of 1988 (OAPCA).
Green Chemistry:An Environmentally Preferable Antifoulant
During the past 10-15 years efforts have been underway to develop antifoulants which are less harmful to the environment but with the same efficacy as organotins. Ideally these compounds should:
- rapidly degrade in the environment and rapidly partition to the sediment, resulting in
-limited bioavailability
- at environmental concentrations be toxic only to target organisms
- result in minimum bioconcentration
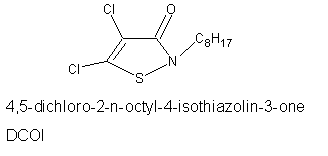
of antifoulants. The effectiveness of DCOI is illustrated by the test panels in Figure 1. Each of the test panels has been immersed in seawater for seven months. The panel on the left has been treated with DCOI and is virtually free of marine organisms, while on the untreated panel (right) the gorwth/attachment of foulants is clearly evident.
Figure 1
Test Panels Immersed for Seven Months in Seawater
Coated with DCOI Not Coated with DCOI
Rohm and Haas Rohm and Haas
DCOI works by maintaining a hostile growing environment for marine organisms. When organisms attach to the hull (treated with DCOI), proteins at the point of attachment with the hull react with the DCOI. This reaction with the DCOI prevents the use of these proteins for other metabolic processes. The organism thus detaches itself and searches for a more hospitable surface on which to grow.
Reducing the Environmental Risk with Sea-Nine 211
Environmental Risk is a function of toxicity and exposure:
ENVIRONMENTAL RISK=f(TOXICITY and EXPOSURE)
Environmental risk can thus be mitigated by reducing the toxicity of a compound and/or by reducing the exposure to the compound. DCOI, the active ingredient in Sea-Nine, by necessity is acutely toxic to a wide range of marine organisms. DCOI was found to be active against algae and diatoms at concentrations of around 10 ppb and barnacle larvae at ppm levels.1 However the environmental risk of DCOI is minimal because only organisms in contact with the ship's hull are exposed to toxic levels of DCOI. Although DCOI is stable as part of the coating on a ship, when it is released (leached) slowly from the hull of the ship it rapidly degrades (when in contact with seawater) to compounds that are essentially nontoxic. This decomposition is a result of microorganisms naturally present in the seawater. The half-life of DCOI in water at pH 7 is greater than 720 hours while in natural seawater the half-life drops to less than an hour1(remember the half-life of TBTO in seawater is >6 months). This rapid biodegradation leads to environmental concentrations of DCOI that are below the acute toxicity level. DCOI is also not chronically toxic, unlike TBTO.
The rapid biodegradation of DCOI is accompanied by rapid partitioning of DCOI to the soil/sediment. This is in part due to the low solubility of DCOI in water. The adsorption of DCOI by the soil/sediment further decreases its bioavailability. In addition to the low bioavailability of DCOI it has a bioconcentration factor of only 13 as compared to 1500 for TBTO.1 Bioconcentration levels of 100 or more are generally considered to be of significance, thus bioconcentration of DCOI is not expected to reach levels in marine organisms that would lead to concern.
In order to calculate the environmental risk of a substance scientists often consider the ratio of the predicted environmental concentration (PEC) of a compound and the concentration of a compound in the environment at which predictions indicate there will be no effect on an organism(s) (predicted no-effect concentration, PNEC). The ratio of the PEC/PNEC is know as
RQ=PEC/PNEC
the risk quotient (RQ) and as the risk quotient gets smaller the less the risk there is associated with the substance. Of course the RQ of a substance should be <1. The RQ of DCOI ranges from .024 to .36 indicating that environmental concentrations of DCOI should pose little risk of toxicity to marine organisms. Conversely the range of RQ for organotin antifoulants is 15-430 indicating a significant risk to marine life.
Questions
1. Describe what is meant by hard and soft foulants.
2. What are the economic consequences of not using an anitfoulant on the hull of a ship? What are the environmental consequences?
3. What is the structure of TBTO?
4.Compounds which have a tendency to bioconcentrate in organisms are relatively nonpolar and degrade rather slowly (persistent). Is TBTO nonpolar (explain your answer)?
5.Why do nonpolar compounds have a greater tendency to bioconcentrate than polar compounds?
6. Are there laboratory methods (that do not use living organisms) that can be used to quantatively predict the tendency of a substance to bioconcentrate (explain your answer)?
7. Why do compounds that are nonpolar but degrade rapidly in the enviroment, not bioconcentrate to any apprecialble extent?
8. What is the structure of DCOI?
9. From the sturture of DCOI would you expect it to be very polar (explain your answer)?
10. Based on your prediction of the polarity of DCOI (in question 9) would you predict that DCOI would have an appreciable tendency to bioconcentrate (explain your answer)?
11. Does DCOI bioconcentrate? Explain why it does or does not bioconcentrate.
12. Would you expect the final product shown in the decomposition of DCOI to bioconcentrate (explain your answer)?
13. What are PEC and PNEC? Explain how PEC/PNEC supplies a measure of risk.
14. If both TBTO and DCOI are placed in seawater at the same concentration how long would it take for each of them to decline to 1/16 of their original concentration.
15. According to the Organotin Antifouling Paint Control Act of 1988 what realease rate of organotin compounds is acceptable? Is the use of organotin antifoulants on all ships prohibited by this act?
16. Describe the difference between the terms acute toxicity and chronic toxicity? Are TBTO and DCOI acutely toxic or chronically toxic (explain your answer)?
17. The technologies nominated for the Presidential Green Chemistry Challenge Awards must fall under one (or more) of three different categories. What are these three categories? Which category does Sea-Nine best fit under?
18. The "Twelve Principles of Green Chemistry" porvide guidelines for developing green chemistry. Which of these twelve principles does Sea-Nine embody?
References and Notes
1. a) https://www.rohmhaas.com/seanine/index.html
b) The Rohm and Haas Company, Designing an Environmentally Safe Marine Antifoulant, a proposal submitted to the
Presidential Green Chemistry Challenge Award Program, 1996.
2. Rouhi, A. Maureen; The Squeeze on Tributyltins. Chem. Eng. News. April 27, 1998, 41-42.
3. https://www.imo.org/briefing/1998/fax04.htm