General Module
Greening Across the Chemistry Curriculum English | Versión en Español
| Versão em Português (Brasil) 
Design and Application of Surfactants for Carbon Dioxide; Making Carbon Dioxide a Better Solvent in an Effort to Replace Solvents that Damage the Environment
Donna Narsavage-Heald, Chemistry Department, University of Scranton
Volatile organic compounds (VOCs) and halogenated organic compounds are used by a wide range of industries for various types of cleaning. Some examples of industrial types of cleaning that employ these compounds as solvents are:
In addition, the individual consumer uses many household products that contain VOCs and halogenated organics. Examples include:
- flux removal
- oil and grease removal from metal parts
- garment cleaning
The worldwide use of VOCs and halogenated organics is estimated to be more than 30 billion pounds per year! Many of these solvents pose a threat to our air quality, water supply, the ozone layer, and our personal health. Briefly, lets explore some examples of these solvents, what they are used for, and the effect they have on our environment.
- stains and varnishes
- paint thinner
- fingernail polish remover
- adhesives
- furniture polish
- hair spray
Volatile Organic Compounds (VOCs)
Figure 1. Sources of VOCs (from epa web site)
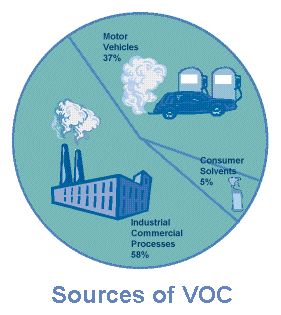
VOCs include solvents such as xylene, toluene, benzene, methylene chloride, chloroform, and isopropyl alcohol. They are widely used in industry because they are relatively non polar and are therefore effective in dissolving oil, wax, and grease. Additionally, the average consumer uses many VOCs on a daily basis. VOCs are also present in gasoline, paints, glues, varnishes, and household cleaning products. VOCs are released from burning fuel (gasoline, oil, wood coal, natural gas, etc.), and automobiles are a major source of VOCs.
These compounds are called volatile organic compounds because they have a high vapor pressure and thus evaporate readily at room temperature. Their high vapor pressure is generally a result of the weak intermolecular forces between these nonpolar molecules. Because of their volatility, they can be used to clean a delicate item such as electronic components or a wool sweater without the use of heat to evaporate the solvent. Also because of their volatility, they can easily escape into the atmosphere and lower air quality.
VOCs react with nitrogen oxide in the presence of sunlight to form ozone (O3) and other pollutants (Scheme 1). Ozone is the principal component of photochemical smog and pollution in many of the world's cities. Additional pollutants that are produced from the interaction of sunlight with VOCs and nitrogen oxide, include nitric acid, which leads to acid rain, and aldehydes, ketones and other compounds resulting from partial oxidation of the VOCs.
Figure 2. Earth's Atmosphere (from epa web site)
Although there is great concern today about depletion of the statospheric ozone layer (Figure 2), to the contrary, the production of ozone in the troposphere poses a significant health risk. The troposphere comprises the atmosphere we live in and the concern with the presence ozone is that there is too much of it in the air that we breathe. Frequent exposure to ozone can result in permanent damage to the lungs, chest pain, coughing, congestion, and nausea. Tropospheric ozone even damages plant life and makes them more susceptible to insects, disease, and other pollutants. Click here to learn more about ozone levels where you live.
Halogenated Organic Compounds
Halogenated organics are carbon based compounds that contain halogen atoms such as fluorine, chlorine, and bromine. Two of the major classes of halogenated organic compounds are the chlorofluorocarbons (CFCs) and the hydrochlorofluorocarbons (HCFCs). These compounds are in general chemically unreactive, nontoxic and nonflammable. These properties have contributed to the popularity of the use of CFCs and HCFCs as solvents for industrial cleaning. In addition these substances have been widely used as refrigerants, propellants for aerosols and blowing agents. The names and formulas of some common CFCs and HCFCs are shown in the table below.
trichlorofluoromethane |
monochlorodifluormethane |
dichlorodifluoromethane |
dichlorotrifluoroethane |
Although CFCs have properties that make them excellent solvents, and useful in many other applications, they are now widely known to decompose in the stratosphere under the influence of high energy UV radiation (UV-C). These decomposition products catalyze reactions that deplete the stratospheric ozone layer, resulting in significant increases in the intensity of harmful UV radiation reaching the surface of the earth. In view of this, under the Clean Air Act of 1990, CFCs can no longer be produced or imported into the United States. HCFCs are being used as temporary replacements for CFCs because HCFCs do not have as great an ozone layer depleting potential. The carbon hydrogen bond in HCFCs makes them much more reactive than CFCs and the vast majority of the HCFC molecules are destroyed in the troposhere. This prevents most of the HCFC molecules from rising into the stratosphere where they too would act to deplete the ozone layer.
Green Chemistry
Carbon Dioxide: An Alternative Solvent that is Environmentally
Preferable to VOCs and Halogenated Organic Compounds
Because of the many negative environmental effects of VOCs and halogenated solvents, finding alternatives to these solvents is important. Carbon dioxide pesents an option to the VOCs and halogenated solvents and offers many advantages in its use as a solvent. Carbon dioxide is nonflammable, nontoxic, and chemically unreactive and it is also available as a cheaply recovered byproduct from the production of ammonia and from natural gas wells.1 In addition, the used carbon dioxide can easily be recovered, purified, and reused.
Unlike CFCs and HCFCs, carbon dioxide does not contribute to the depletion of the ozone layer, and unlike VOCs, carbon dioxide is not a factor in the creation of tropospheric ozone. However, carbon dioxide has other negative environmental effects. Because carbon dioxide in the atmosphere reflects infrared radiation (heat) back toward the earth, it is a greenhouse gas and it is thus a contributor to global warming. In order for CO2 to be considered as a green alternative to VOCs and halogenated organic solvents, the used CO2 should be recycled. After using carbon dioxide as a solvent it can be recovered and reused by simply allowing the supercritcal or liquid carbon dioxide to be converted to a gas, capturing the gaseous carbon dioxide, and leaving the less volatile impurities behind. This recycling process requires very little energy since CO2 exists as a gas under ambient conditions. Compared with organic solvents or water, its enthalpy of vaporization is considerably lower.
Another consderation when assessing carbon dioxide as a replacement for less environmentally friendly solvents, is the source of the CO2. As indicated previously, carbon dioxide is produced as a waste product of ammonia production and from natural gas wells and can be recovered from these processes. Thus, no additional carbon dioxide need be produced, and the carbon dioxide that would normally be vented into the atmosphere from these processes, can actually be captured and put to good use. Many of these factors contribute to carbon dioxide being a green alternative to VOCs and halogenated organic compounds.
As indicted above carbon dioxide is a gas at room temperature and atmospheric pressure. In order to use CO2 as a solvent, it must be converted into a supercritical state or a liquid state. As illustrated in the following phase diagram, carbon dioxide has a supercritical temperature (Tc) of 31 °C and a supercritical pressure (Pc) of 72.8 atm. Supercritical fluids have properties of both a gas and a liquid. Supercritical CO2 is currently used as a solvent to "naturally" decaffeinate coffee.
Solubility of Substances in CO2
Although carbon dioxide has polar bonds it is a nonpolar molecule since the dipoles of the two bonds cancel one another.

Because of this nonpolar nature, carbon dioxide will dissolve smaller nonpolar molecules such as hydrocarbons having less than 20 carbon atoms and other organic molecules such as aldehydes, esters, and ketones,2 but it will not dissolve larger molecules such as oils, waxes, grease, polymers, and proteins, or polar molecules. However by using a surfactant, the solubility of many substances in CO2 can be increased.
Surfactants are substances that increase the solubility of one substance in another. Soaps and detergents are common surfactants that enable nonpolar substances like oil and grease to be emulsified and washed away with the polar solvent water. From our everyday experiences, we know that oil and water do not mix, that is they are insoluble in each other. They are insoluble in each other because oil is nonpolar and water is polar and the rule of thumb for solubility is "like dissolves like". In other words, polar solvents disolve polar solutes, and nonpolar solvents dissolve nonpolar solutes. A surfactant is a molecule that contains a polar portion and a nonpolar portion. By having both polar and nonpolar groups within the same molecule, a surfactant can interact with both polar and nonpolar molecules, thereby increasing the solubility of two otherwise insoluble substances.
In water, surfactant molecules tend to cluster into a spherical geometry with their nonpolar ends on the inside of the sphere and their polar ends on the outside. The polar ends are on the outside so they can interact with the polar solvent water. These clusters are called micelles and an example is shown in Figure 4.
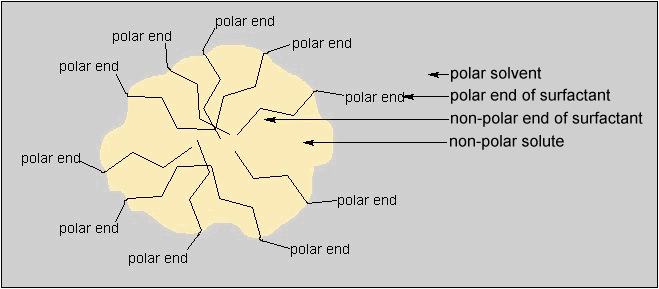
Figure 4. Micelle Structure of a Surfactant in a Polar Solvent
(reprinted with permission from the American Chemical Society)
A surfactant for liquid or supercritical fluid CO2 will have to have both CO2-philic (CO2 loving) and CO2-phobic functionality. In 1994, Joseph M. DeSimone of the University of North Carolina and North Carolina State University published his discovery that polymers such as those shown in Figure 5 are soluble in liquid or supercritical fluid CO23. This fluoropolymer is soluble in CO2 because of the weak van der Waals attractive forces that are present between CO2 and the fluorocarbon tail of the polymer.
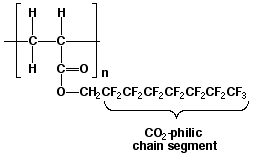
Figure 5. Polymer with CO2-philic Fluorocarbon Tail
Polymers are molecules with very high molar masses. Water has a molar mass of 18 amu, but polymers typically have molar masses of 10,000 up to several million amu. Polystyrene is a very common polymer and has the repeat unit:
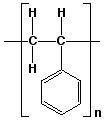
Figure 6. Polystyrene
This means that the unit contained within the brackets repeats over and over again. More specifically, it repeats n times and we would call n the degree of polymerization. For most polymers, n > 1000.
Polystyrene is used for a variety of packaging applications, such as plastic drinking cups, plastic cup lids, packaging for small produce items such as berries or cherry tomatoes. As a foam, it is commonly known as styrofoam and is used as a drinking cup for hot beverages, foam insulation, and the familiar 'peanuts' used to protect delicate items in shipping.
Polystyrene is a brittle polymer and is most often combined with other polymers to improve its properties. One way to modify the properties of a polymer is to make a copolymer. A copolymer contains two different types of repeat units within the same polymer chain. A copolymer is not a blend of two different polymers, but instead the two monomers are covalently bonded along the length of the chain. A copolymer of styrene and acrylonitrile is used to make automobile parts, appliances (refrigerator shelves), medical supplies, and battery cases.
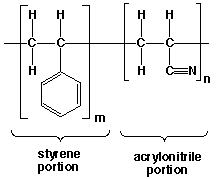
Figure 7. Styrene Acrylonitrile Copolymer
Copolymers can exist in a variety of arrangements. Three possible copolymer sequencing arrangments are shown below. In these structures, S represents the styrene monomers and A represents the acrylonitrile monomers.
The polymer shown in Figure 5 is unusual because most polymers are not soluble in CO2. In fact, the polymer in Figure 5 is one of the only two CO2 soluble high molar mass polymers4. So, to make a CO2 surfactant, DeSimone synthesized copolymers using polystyrene as the CO2-phobic portion and the polymer shown in Figure 5 as the CO2-philic portion2. Figure 8 shows the structure of this block copolymer. The copolymer shown in Figure 8 can be schematically represented as SSSSSSSSAAAAAAA, where S=styrene units and A=fluoroacrylate units.Random SASASAASASSAS
Block SSSSSSAAAAAAA
Alternating SASASASASASASA
Figure 8. Copolymer Containing CO2-phobic and CO2-philic Units
Just as surfactants for water form micelle structures, so do surfactants for CO2. Figure 9 shows this micelle structure for a CO2 surfactant, with the CO2-philic portion on the outside of the micelle. As with detergents that act as surfactants for cleaning using the solvent water, a CO2 surfactant can increase the solubility of greases, waxes, and oils in the solvent CO2.
Figure 9. Micelle Structure for CO2 Surfactant
(reprinted with permission from the American Chemical Society)
Current Use of CO2 Surfactants--Green Chemistry in ACTION
The dry cleaning industry typically uses the solvent perchloroethylene (PERC), as the cleaning agent. Because clothes are cleaned in a liquid solution that is mostly PERC, and very little if any water is used, the term "dry cleaning" is used.

Figure 10. Tetrachloroethylene or Perchloroethylene (common name)
It is estimated that 344 million lb of PERC were produced in the United States in 1998. The dry cleaning industry uses approximately 50% of the PERC produced each year, which amounts to 172 million pounds of the solvent. The EPA has classified PERC as a groundwater contaminant and a potential human health hazard. PERC is a suspected human carcinogen and a known rodent carcinogen. Breathing PERC for short periods of time can adversely affect the central nervous system. Effects include dizziness, fatigue, headaches, sweating, and possibly loss of coordination and unconsciousness. These effects are not likely to occur though at levels of PERC that are normally found in the environment, but people who work in the dry cleaning industry have the greatest risk for exposure.
The use of PERC in the dry cleaning industry began in the late 1930's. Prior to PERC, petroleum based products such as kerosene were the dry cleaning solvent of choice, but because of their high flammability, they were hazardous to work with and fires were common. PERC however, is not flammable and thus offers a significant advantage over petroleum based cleaners. Because of the double bond in PERC, it is destroyed in the troposphere preventing it from rising to the stratosphere. Thus, unlike many other halogenated organic compounds, PERC does not contribute to the destruction of the ozone layer. However, PERC can contribute to the formation of photochemical smog, when it reacts with other VOCs, nitrogen oxide, and sunlight (Scheme 1).
Micell Technologies, a company founded in 1995 by Joseph DiSimone, Timothy Romack, and James McClain, has made the CO2 surfactant technology available commercially. Micell's Micareô system is a commercial washing machine that utilizes CO2 and a CO2 surfactant instead of PERC, thereby eliminating the need for the chlorinated hydrocarbon, PERC. The francise, Hangers, uses this technology.
Micell Technologies also developed technology known as the Micleanô system for cleaning oils and greases from metal components, once again eliminating the need for halogenated cleaning solvents.
Professor DiSimone won a Presidential Green Chemistry Challenge Award in 1997 for his discovery and development of the carbon dioxide surfactants. He was also awarded the Governor's Award for Excellence, the National Science Foundation's Young Investigator Award and the Presidential Faculty Fellow Award. He and Micell Technologies also received the R&D 100 Award when their Micareô drycleaning system was recognized as being one of the "100 most technologically significant new products and processes of the year."
References
1. DeSimone, Joseph M. Design and Application of Surfactants for Carbon Dioxide, a proposal submitted to the Presidential Green Chemistry Challenge Awards Program, 1997.
2. Hyatt, John A. Liquid and Supercritical Carbon Dioxide as Organic Solvents. J. Am. Chem. Soc. 1986,49, 5097-5101.
3. Guan, Zhibin; DeSimone, J.M. Fluorocarbon-Based Heterophase Polymeric Materials. J.Am. Chem. Soc. 1994, 27, 5527-5532.
4. DeSimone, J.M.; Maury, E,E.; Menceloglu, Y.Z.; McClain, J.B.; Romack, T.J.; Combes, J.R. Dispersion Polymerizations in Supercritical Carbon Dioxide. Science, 1996, 271, 624-626.