A Green Chemistry Module
Suggested Use:An advanced organic chemistry course during a discussion of nucleophilic substitution reactions or aromatic substitution reactions.
trudy.dickneider@scranton.edu
Introduction
Substituted benzene molecules have tremendous importance in industrial chemical applications. They are commonly used as solvents and they are often important intermediates in many syntheses, including those of powerful pharmaceutical agents.One class of compounds produced in this way serves as intermediates in the synthesis of antidegradants compounds that are very important in the chemical rubber industry.This module deals with a recently developed method for the production of one of these intermediates, 4-aminodiphenylamine (4-ADPA). The chemistry presented exemplifies an innovative approach to the problem of introducing nucleophiles onto aromatic ring systems in a way that greatly reduces the negative environmental problems associated with the more well known synthetic procedures.
The most common method of producing substituted benzene rings involves the well-known reaction of electrophilic aromatic substitution (EAS).This allows facile substitution for hydrogen of nitro groups, halogens, sulfonic acid, alkyl and acyl groups.While the applications of this reaction for multiple substitutions are limited by the ortho/para or meta directing properties of groups on the ring as well as their activating/deactivating properties, it is usually possible to prepare the desired compound as long as the group to be introduced to the ring is an electrophile.This means that a whole family of substituents, which are nucleophilic in nature, cannot be introduced to the ring by EAS. In general, reactions involving the substitution of nucleophiles are difficult. Under the usual conditions for nucleophilic substitution reactions they produce vanishingly small amounts of product.Recalling the two mechanisms for nucleophilic substitution, SN1 and SN2 demonstrates the reason: with an aromatic ring there is no available backside approach to the carbon, hydrogen is a very poor leaving group, and the aromatic system will not readily ionize by loss of hydrogen.Therefore, in order to introduce important nucleophiles onto the aromatic ring some tricks have to played on the ring itself. Under the special conditions which then result it is possible to incorporate nucleophiles onto the ring. It is this reaction, Nucleophilic Aromatic Substitution (NAS) that is the topic of this module.We will examine the traditional chemistry for NAS along with the elucidated mechanisms.In doing this we will see that this chemistry has several environmental problems that make it undesirable as a large scale industrial process.In response to these problems Flexsys America L.P. (1) developed a method for eliminating these problems in the production of the industrially important intermediate, 4-aminodiphenylamine. In recognition of their work they were awarded a Presidential Green Chemistry Challenge Award.We will examine the mechanism and the conditions for this reaction and speculate on its adaptability to further syntheses.
The Mechanisms
There are at least four mechanisms (2), operating under different conditions, for the introduction of a nucleophile onto an aromatic ring.They are the SNAr, Benzyne, SN1, and SNR1 mechanism.Although these four have been identified and studied most nucleophilic aromatic substitution reactions which are commonly performed are accomplished by either the SNAr or Benzyne mechanisms so we will concentrate on those and only briefly consider the other two.
SNAr mechanism [addition/elimination]
This is the most important of the mechanisms for nucleophilic aromatic substitution reactions. The experimental evidence indicates that there are two steps and so it is often referred to as the addition/elimination mechanism. The reaction begins with attack by the nucleophile on the ring carbon bearing the leaving group. This is the addition step of the reaction. This produces an anionic intermediate. This ion, known as the Meisenheimer complex, is resonance stabilized.
In the second step of the reaction the elimination occurs as the ring undergoes rearomatization with the loss of the Leaving Group. The first step, in which the ring loses it aromatic stabilization and the anion is produced is the slow or Rate Determining Step (RDS).
However, the reaction as shown above would not proceed to yield product!Resonance stabilization alone is not sufficient for the anionic intermediate to form. It must be stabilized by strong electron-withdrawing groups in positions ortho and/or para to the leaving group.
The most important electron-withdrawing group(G) for these reactions is the Nitro group (-NO2). It will activate the ring for NAS reaction with strong nucleophiles at moderate temperatures (80-100oC). There are several other Groups that will activate the ring for room temperature reaction with strong nucleophiles when the nitro group is present in either the ortho or para position.
Mechanisms are, of course, based on experimental evidence. There are several important observations and results that support the SNAr mechanism.The most important of these is the isolation of the intermediate, surely the most persuasive pieces of evidence for any mechanism!
The reaction shown below produces a stable salt which has been isolated and characterized. It was first isolated in 1902 by Jacob Meisenheimer (3) who proposed the correct structure which was later confirmed by NMR studies.
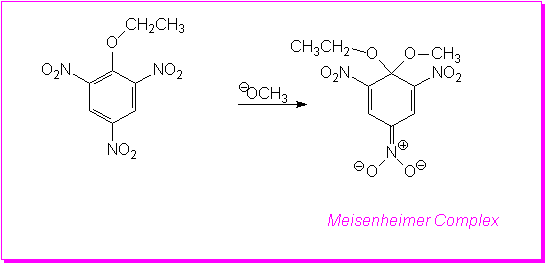
Further support for the SNAr mechanism comes from the fact that the nature of the Leaving Group has little effect on the course of the reaction.Like all anionic leaving groups, the LG in the NAS reaction leaves with a pair of electrons so it must be a species that is able to bear a negative charge.However, since only small effects are seen upon varying the LG in comparing species which form stable anions, it would indicate that the bond to the Leaving Group is not broken in the rate determining step of the reaction. This supports the two step nature of the SNAr mechanism.For the halogens, which are the most common leaving groups in NAS, their reactivity parallels their electronegativity ( F>>Cl, Br, I) since the more electronegative the atom, the more electron density it withdraws from the carbon. The more positive the carbon, the faster the attack by the nucleophile will be.
This reaction has found great synthetic utility, however, it produces a product with the necessary activating electron-withdrawing groups attached to the ring! When the NAS reaction is part of a multistep synthetic scheme, that may or may not be desirable.Therefore, NAS reactions based on this one mechanism alone will not allow synthesis of all desired products.
The Benzyne Mechanism [elimination/addition]
It is possible for a benzene ring bearing a good leaving group to undergo Nucleophilic Aromatic Substitution without strongly electron-withdrawing groups substituted in the ortho/para position(s).For this to happen the aromatic substrate must be treated with a base strong enough to abstract a proton.When the Base/Nucleophile abstracts the hydrogen, the sigma electrons bonding the hydrogen to the carbon are then contributed to the carbon bearing the leaving group, causing the departure of the leaving group. This produces a unique intermediate, BENZYNE. This intermediate then reacts with the conjugate acid of the Base/Nucleophile, which adds the nucleophile and returns the ring to its original aromatic state.
The overall result is that in this reaction the order of the processes is reversed compared to the SNAr mechanism; the benzene substrate undergoes an elimination reaction – loss of hydrogen and LG- followed by an addition of the nucleophile.
There is strong experimental support for the Benzyne Mechanism.The first piece of evidence rests on leaving group ability.For the halogens, again the most important leaving group, the order(Br > I > Cl >> F) deviates significantly from that seen in the SNAr mechanism. (4) This indicates that a different mechanism is operating.
The identity of the leaving group also affects which step is rate determining, another piece of supporting evidence.To understand this requires a closer look at the Elimination step of this mechanism. This step may be concerted (as implied above) or it may be stepwise (see below).
If the elimination is stepwise, when the LG is Br or I the first step, the loss of hydrogen, is the RDS. However, when the LG is Cl or F, the loss of the LG is rate determining.
Another important clue to the nature of this reaction is given by the fact that in substrates where there are substituent groups in both ortho positions to the Leaving Group there is no reaction, since the Benzyne intermediate could not form. (4)
Studies of reaction mechanisms often benefit from the use of substrates labeled with radioisotopes. An interesting study of this mechanism was performed using a 14C labeled compound. (5)
The most convincing evidence for this mechanism, however, might be that associated with the Benzyne intermediate itself. While it has never been isolated under experimental conditions it has been studied in argon at 8 K and its spectrum has been recorded during the progress of reactions. (6)
The available electron density of the “triple bond” makes it a pseudo-dienophile in a Diels-Alder reaction.When Benzyne in generated following diazotination of anthranilic acid in the presence of a Diene, such as furan, a Diels/Alder adduct is recovered from the reaction mixture.(7)
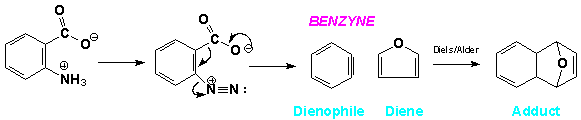
This trapping of the intermediate Benzyneis strong evidence supporting its existence.
Other Mechanisms
There are two other mechanisms possible for nucleophilic aromatic substitution. They each deal with specialized substrates and therefore, are not common routes to aromatic rings substituted with nucleophilic species. Therefore, we will examine them only briefly, concentrating on the reaction and some evidence for each route proposed.
SN1 Mechanism
This is a common mechanism for substitution of nucleophiles for diazonium salts.In this case the leaving group is not a halide or sulfonate, as in most NAS reactions, but rather the nitrogen molecule lost from the diazonium salt.
The most important evidence for this reaction mechanism comes from kinetic studies that indicate that the reaction is first order in diazonium salt, so the slow step of the reaction is the decomposition of the salt.Since the nucleophile is not involved in the RDS, the reaction is actually an SN1 reaction for aromatic substitution.
SNR1 Mechanism
There is experimental evidence that indicates that certain aromatic substrates, particularly iodobenzenes, undergo nucleophilic substitution reactions by a free radical mechanism. The proposed mechanism for this reaction begins with an initiation step in which an electron is transferred to the aromatic substrate from an electron donor, forming a radical anion. This species loses iodide in the first propagation step to give an aryl radical. The nucleophile reacts with the aryl radical to give another radical anion in the second chain propagation step. In the third step of the chain this radical loses an electron to another molecule of substrate producing the nucleophilic substitution product and another radicalanion, thus establishing the chain that is characteristic of free radical reactions. The chain propagation steps are followed by several types of termination steps, including combinations of radicals formed in the propagating steps or the reaction of the aromatic radical with a hydrogen donor to produce benzene.
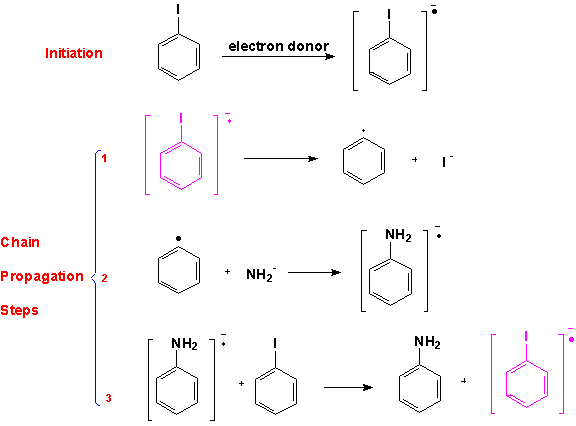
One of the most important pieces of experimental evidence for this mechanism is the fact that no reaction takes place when radical scavengers are introduced into the reaction mixture.Also, this mechanism explains observed substitution patterns of nucleophilic aromatic substitution reactions that are inconsistent with expectations from either the SNAr or Benzyne mechanisms. An example of these results is shown below for the reactions of a 1,2,4-trimethylbenzene substituted in the 5 and 6 positions.(8)When the substituent was chlorine or bromine the NAS product was the expected 5 and 6-substituted product in roughly equal amounts. These would, of course, be the expected results from the Benzyne mechanism since either product would be equally likely from the Benzyne intermediate.However, when the trimethyl benzene was substituted with iodine ins the 5 and 6 positions, products with the nucleophile in the 5 and 6 positions were not obtained in equal amounts indicating that another mechanisms was operating with the iodobenzene substrate.
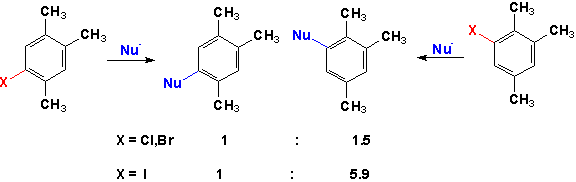
The SNR1 mechanism has been found to be very versatile since no activating groups are needed as substituents on the ring for reaction to take place. The reaction can be initiated electrochemically or photolytically and substrates containing the other halogens as well as a quaternary methyl amine will also undergo reaction by the free radical mechanism in the presence of an electron donor to initiate the reaction.
Green Chemistry - An alternative synthetic pathway for
Aromatic Nucleophilic Substitution
Green Chemistry Challenge Award -Elimination of Chlorine in the Synthesis of 4-Aminodiphenylamine: A New Process Which Utilizes Nucleophilic Aromatic Substitution for Hydrogen
Introduction
The chemical rubber industry uses several classes of compounds as antidegradants. They are antioxidants and antiozonants.These compounds serve an important purpose because they protect the rubbers, natural or synthetic, from the harmful effects of exposure to the atmosphere and sunlight.The types of organic compounds used as antidegradants are shown to the left.The antioxidants slow the oxidation of the rubber. They react with hydroperoxides and produce compounds which do not react with the rubber. Since it is thought that rubber cracks as a result of an oxidative process which hastens any mechanical cracking, they also serve an antiflex purpose and keep the rubber from cracking. Some are also classified as antifatigue agents. The antiozonants are used in combination with unsaturated rubbers to prevent reaction with ozone from the atmosphere which causes surface cracking in the rubber compound.While the mechanism for the action of the antiozonants is not well understood, they apparently function by coating the rubber surface. One of the most important intermediates in the synthesis of these antidegradants is 4-aminodiphenylamine (4-ADPA).
The Existing Industrial Method
The current industrial preparation of 4-ADPA uses a two electrophilic aromatic substitution reactions in order to prepare the ring for nucleophilic aromatic substitution. The first, chlorination of benzene provides the leaving group. The second, nitration of the chlorobenzene, serves to activate the molecule for nucleophilic aromatic substitution reaction needed to add formanilide to the substrate. Hydrogenation of the resulting diphenyl nitrate compound yields the desired 4-aminodiphenylamine.
The sequence begins with a well-known electrophilic aromatic substitution (EAS) reaction in which the chlorine atom is substituted for hydrogen on the benzene nucleus.Another EAS reaction nitrates the compound in the ortho and para positions. This regioselectivity results from the directing nature of the chlorine present on the substrate.It is electron donating by resonance which stabilizes the transition state species leading to the intermediate benzenonium ion for the ortho and para products. Recall, that although the halogens are electron releasing through resonance, they are electron-withdrawing by induction and the inductive effect is stronger than the resonance effect. Therefore, this reaction would proceed at a slower rate than nitration of unsubstituted benzene. These EAS reactions are shown below.
The para-nitrochlorobenzene (PNCB) produced in the EAS reaction is the substrate for the nucleophilic aromatic substitution reaction. This reaction proceeds according to the SNArmechanism we have discussed above.In this case the lone pair electrons on the nitrogen of the formanilide act as the nucleophilic electron density.The product of the first step of the sequence is 4-nitrodiphenylamine (4-NDPA). A catalytic hydrogenation yields the synthetic goal, 4-ADPA.
The Atom Economy of the Reaction
Chemists have traditionally measured the efficiency of a reaction by determination of the percentage yield (% yield) of the reaction. However the % yield deals with only the amount of the desired product(s) that is isolated (relative to the limiting reagent) and does not consider the efficiency of the reaction relative to (waste) byproducts that are formed.The concept of Atom Economy (developed by Barry Trost) extends the consideration of the efficiency of a reaction to take into account all the atoms in the reactants and whether these are incorporated or utilized in the desired product or whether they end up (unutilized) in byproducts.One can calculate the % Atom Economy determining the mass of all the atoms that are utilized in the desired product, dividing this mass by the total mass of all the reactant atoms and multiplying by 100. For a more complete discussion see the Green Module for Organic Chemistry: “
ATOM ECONOMY: A Measure of the Efficiency of a Reaction”
An examination of the reactions above demonstrates several atom economy problems. The first EAS uses two chlorine atoms, neither one of which ends up in the product.
The overall reaction scheme for the traditional preparation of 4-ADPA is shown below. The substrates and reagents are labeled in red.
The calculation of the Atom Economy is shown in the table below.
Reagent formula
|
Reagent FW
|
Utilized Atoms
|
Wt
|
Unutilized Atoms
|
Wt
|
1 C6H6
|
78 |
6 C, 4 H
|
76 |
2 H
|
2 |
2Cl2
|
70 |
------------
|
0 |
2 Cl
|
70 |
3 HNO3
|
63 |
1 N
|
14 |
1 H, 3 O
|
49 |
4 C7H7NO
|
121 |
6 C, 6 H, 1 N
|
92 |
1 C, 1 O, 1 H
|
29 |
5 K2CO3
|
98 |
------------
|
0 |
2 K, 1 C, 3 O
|
98 |
6 H2
|
2 |
2 H
|
2 |
--------------
|
0 |
TOTAL
|
432 |
12C, 12H, 2N
|
161 |
2C,4H,2Cl,2K,7O
|
248 |
The Atom Economy of the reaction is equal to the weight of utilized atoms divided by the weight of all reagents used.
% Atom Economy =161/432 x 100 = 37%
This calculation demonstrates clearly the problems associated with the current chemistry.
Brown Chemistry of the Current Reaction
Besides the unfavorable atom economy and the resulting environmental as well as economic impact, the reactions currently used to prepare 4-ADPA present several other environmental concerns. The first is the % yield of the desired para-nitrochlorobenzene is only 70% with the other 30% being the unwanted byproduct, ortho-nitrochlorobenzene. The second is the use of another aromatic hydrocarbon, xylene, as a solvent for the NAS reaction.This material would have to be properly disposed of as toxic chemical waste presenting a potential environmental hazard and adding to the cost of preparing the 4-ADPA.The major concerns about this reaction pathway, however, center around two aspects. The first, and most important, is the fact that large amounts of chlorine gas are used in the first step of the reaction. This gas would have to be stored and handled properly to minimize the risk of escape to the atmosphere where it would have severe environmental and health consequences. Also it is a SARA (Superfund Amendments and Reauthorization Act) list compound and the amount of chlorine used and stored must be reported annually to the EPA. Plants utilizing the SARA materials must also file a hazardous materials plan demonstrating their planning for dealing with spills and accidents involving the compound. The second concern is the amounts and variety of wastes produced in this reaction sequence.The total pathway produces an aqueous solution of inorganic salts.The problem is that this aqueous waste stream would be contaminated with the organic materials which are the substrates and products of the steps in this reaction sequence since they are all polar enough to have some solubility in water. While it is certainly not impossible to separate the wastes from this reaction it is time-consuming, expensive, and presents more risks of contamination of the environment.The nature of this chemistry is shown in the fact that Monsanto Chemical Company considered this reaction “one of the most wasteful and potentially dangerous” reactions that they performed in their various operating plants! (9)
It was clear that the solution was to design a reaction that could yield the 4-ADPA without using a chlorinated starting material.
The Green Chemistry Alternative
Development of the Reaction
The research and development chemists at Monsanto realized that the solution to these environmental problems involved replacing the traditional NAS reaction with one that involves substitution for hydrogen, thereby eliminating the need for the chlorinated substrate.There were several examples of these nucleophilic aromatic substitution for hydrogen (NASH) reactions known in the literature. The new chemistry was developed by Flexsys America.
The Alternate Reaction Pathway
The general reaction for a nucleophilic aromatic substitution for hydrogen reaction is shown below.
The application of this reaction to the synthesis of 4-ADPA was developed by the work of Sterns, Hileman, and Bashkin.(10)The new chemistry involves the coupling of aniline and nitrobenzene in a reaction that is a substitution for hydrogen.This type of reaction was first reported in the literature in 1903 and proceeded with a 50% yield.
In fact, the NASH reactions in the literature all suffered from the same limitations.As a group they proceed with very low yields and show poor selectivities.While the reaction might be feasibly used to produce compounds in small amounts on a laboratory scale for research purposes, these drawbacks make the reaction unsuitable for industrial applications in the preparation of large amounts of material.
The reaction proposed by the Monsanto chemists uses a base to produce a better nucleophile. The base abstracts a proton from aniline producing an anilide ion, a much more powerful nucleophile.The first step of the reaction, then, is a base promoted coupling reaction in which the anilide ion attacks the carbon para to the activating nitro group.
As shown below the product of this reaction is then oxidized under anaerobic conditions to two intermediate products, 4-nitrosodiphenylamine (4-NODPA) and 4-nitrodiphenylamine (4-NDPA).The first (4-NODPA) is produced by an intramolecular reaction in which the nitro group of the coupled product acts as the oxidant.
In the simultaneous intermolecular reaction (see below) the oxidant is the reactant nitrobenzene. This route yields the 4-NDPA and azobenzene.The azobenzene produced by this oxidation is easily recovered and converted back to aniline so it is not a waste product of the reaction.By optimizing the reaction conditions, the Monsanto chemists achieved an overall 96% yield of the two intermediate products. In addition, their increased understanding of the mechanism of the reaction has led to other applications of nucleophilic aromatic substitution for hydrogen in which amides are reacted with nitrobenzene producing industrially important amines.(11)
Atom Economy of the Flexsys NASH Reaction
A similar calculation of the Atom Economy of the Flexsys NASH reaction is based on the overall reaction shown below.
Again, the data for the reagents and substrates labeled in red are shown in the table below.
Reagent Formula
|
Reagent FW
|
Utilized Atoms
|
Wt
|
Unutilized Atoms
|
Wt
|
1 C6H6
|
78
|
6 C, 4 H
|
76
|
2 H
|
2
|
2 HNO3
|
63
|
1 N
|
14
|
1 H, 3 O
|
49
|
3 C6H7N
|
93
|
6 C, 6 H, N
|
92
|
1 H
|
1
|
4 H2
|
2
|
2 H
|
2
|
-----------
|
0
|
TOTAL
|
236
|
12C,12H,2N
|
184
|
4H,3O
|
52
|
The Atom Economy of the Flexsys NASH reaction is given by
% Atom Economy =184/236 x 100 = 78%
and represents a two-fold improvement over the traditional chemistry!
Green Chemistry of the Flexsys NASH
This alternate reaction sequence has many significant environmental advantages over the traditional route. The company describes five important environmental advantages of the new process.(12) They are:
- ·Tremendous reduction of organic waste from the industrial reaction. The Flexsys chemists report that the new reaction reduces the organic waste by 74% and eliminates 99% of the inorganic waste.In the Green Chemistry Challenge Award Nomination Flexsys states that if only 30% of the worlds’ supply of 4-ADPA were produced by NASH there would be a savings of 74 million pounds of waste.
- ·Reduction of waste water generated by more than 97%. If the Flexsys chemistry were used to generate 30% of the annually produced 4-ADPA it would save1.4 billion pounds of water per year.
- ·Elimination of the use of chlorine, a dangerous chemical and a difficult one to handle.
- ·Elimination of the use of xylene which is a necessary solvent in the traditional chemistry.Xylene, an aromatic hydrocarbon, poses its own set of handling, storage and disposal problems.
- ·Improved industrial safety. Compared to the traditional chemistry to produce 4-ADPA, the new chemistry does not use several process streams and high temperatures, conditions that can and have led to runaway reactions.Monsanto described this synthetic process as one of its most dangerous and they report that they experienced runaway conditions in 1979 which resulted in the explosion of a reactor. The new chemistry does not show any potential for runaway conditions.
Study Questions:
1.Draw the structures of the important resonance contributors for the Meisenheimer complex for the SNAR reaction of the following substrates.
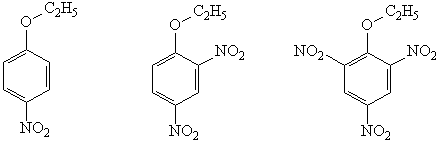
2. Based on the structures you have drawn in question 1, which compound would you predict to be the best substrate for nucleophilic aromatic substitution by the SNAr mechanism? Explain your answer clearly.
3. The Flexsys chemists have extended their NASH chemistry to the synthesis of amines from the reaction of nitrobenzene with amides.Do a literature search to find a reference for a reaction of this type. Compare the proposed mechanism for this reaction to that for the production of 4-ADPA.
4. Draw a Benzyne mechanism for the reaction of both 5-chloro and 6-chloro-1,2,4-trimethylbenzene with amide showing the origin of the 5 and 6 substituted product from each substrate explaining the observed product ratio.
5.What experimental means would you use to determine whether a nucleophilic aromatic substitution reaction was proceeding by an SNAR or Benzyne mechanism?
6. Outline a reaction sequence that would convert azobenzene to aniline.
7. The product of the Flexsys synthesis, 4-ADPA, is an important intermediate in the synthesis of the antidegradants used in compounding rubbers. One of these antidegradants which serves as an antiozonant and antiflex compound is N-isopropyl-N’-phenyl-p-phenylene diamine. Outline a reasonable synthesis of this compound using 4-ADPA.
Suggestions for Further Study
1. Using a molecular modeling program construct a model of benzyne showing the location of the p electron density in the intermediate.
2. The Nanotube Connection, a NASA web site (http://www.ipt.arc.nasa.gov.gallery.html) contains information on the use of benzyne molecules as gears on machines constructed from nanotubes.What is a nanotube ? How are they made? What are the possibilities for machines of the type described on this web site?
References
1.Rains, Roger K., et al., Elimination of Chlorine in the Synthesis of 4-Aminodiphenylamine: A New Process Which Utilizes Nucleophilic Aromatic Substitution for Hydrogen, a proposal submitted to the Presidential Green Chemistry Challenge Awards Program 1998.
2.Comprehensive, detailed discussions of these mechanisms with extensive references are found in:
March, J. Advanced Organic Chemistry, Wiley-Interscience, NY (1992).
Carroll, F.A. Structure and Mechanism in Organic Chemistry, Brooks/Cole, NY (1998).
3.Meisenheimer, J. Liebigs Ann. Chem., 323, 205 (1902).
4.Roberts, J. D., et al., J. Amer. Chem. Soc. 78, 601 (1956).
5.Roberts, J. D., op. cit.
6.Chapman, N. B.. et. al., J. Amer. Chem. Soc., 98, 6710 (1976).
7.Stiles, P. J., and Miller, J., J. Amer. Chem. Soc., 82, 3802 (1960).
8.March, op. cit.
9.Stern, M.K. et al., J. Amer. Chem. Soc., 114, 9237 (1992).
10.Stern, M K., et al., New Journ. Chem., 20, 259 (1996).
11.Stern, M. K. and Cheng, B., J. Org. Chem., 58, 6883 (1993).
12.Rains, op. cit.